Introduction
Metabolism is a term used to refer, virtually, to all the chemical processes taking place in the body. The normal physiological processes defining nutrient modification are controlled by particular glucoregulatory hormones, for example, insulin, glucagon, some adipokines, cortisol, growth hormone, the incretins, and epinephrine. The purpose of this paper is to discuss diabetes mellitus and insulin; hence, insulin will be the main focus. Resistance to insulin and associated biological activities leads to the development of diabetes mellitus because of elevated glucose in the blood. The ability of insulin to reduce blood glucose is known as insulin sensitivity. Diabetes is a chronic disease with a high prevalence, globally, and the disease has continued to escalate over the years. Diabetes is associated with severe complications because of altered metabolic processes, which define normal body functions. This paper, therefore, discusses the structure, synthesis, secretion and metabolism of insulin in association with its major function, which is to regulate blood glucose.
Anatomy of pancreas
The pancreas is a retroperitoneal structure that is finely lobulated. The pancreas is located on the posterior abdominal wall, behind the stomach and various organs, including the ileum, liver and spleen surround it as shown in figure 1 below. Its length is approximately 6 six inches, and it has a shape like that of a flat pear. The pancreas is made up of four parts; however, this varies depending on available literature. The four parts include the insulin head (close to the curve of the duodenum), neck, body (which is the central portion) and a tail. The head and the neck join as the neck passes, anteriorly, over the vertebral column. The gastroduodenal artery lies anteriorly to the neck while the portal vein relates to the neck posteriorly.
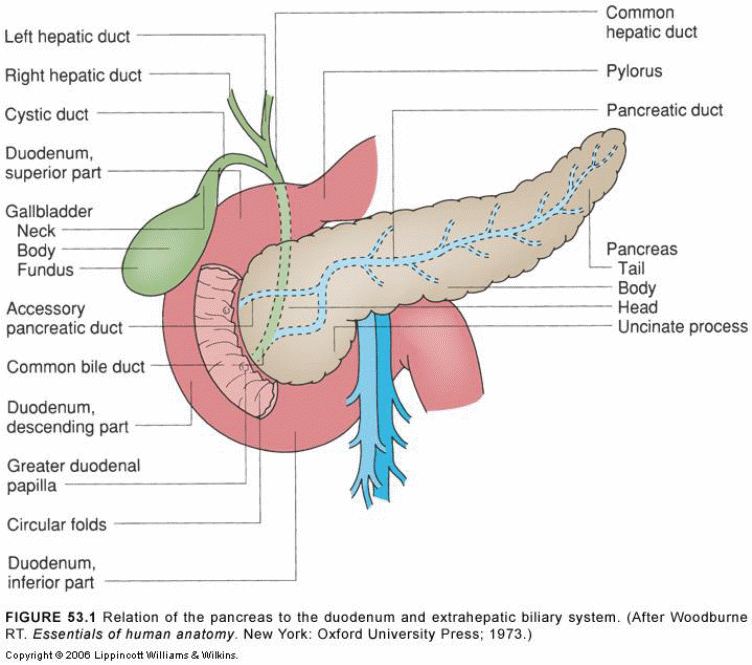
A CT scan mainly shows that the liver has a similar density to the pancreas, but with increasing age, this changes due to progressive accumulation of fat.
The pancreas has two discrete functional and morphological compartments (exocrine and endocrine compartments) emanating from the endoderm. The exocrine compartment makes up 98% of the total pancreas mass and mainly consists of enzyme-secreting acinar cells arranged in clusters around a network system of ducts for transporting secretions into the duodenum. The endocrine system, on the other hand, makes up <2% of the organ mass. This section of the pancreatic tissue is made up of highly vascularized and innervated cell clusters, what is commonly known as the islet cells that comprise of five distinct hormone-secreting cell subtypes (α, β, δ, ε & pancreatic polypeptide).
Unfortunately, exogenous insulin is not able to work effectively as the endogenous insulin due to the pharmacodynamics involved. The first process that insulin undergoes is to enter the liver through the portal vein. Here, more than 50% of the insulin is extracted; thereby increasing hepatic exposure to insulin while lowering muscle and fat (peripheral) exposure to the hormone.1 The situation is reversed when exogenous insulin is given.
Structure of Insulin
The release of insulin from the pancreas depends on blood glucose levels; high levels stimulate production of insulin, and the converse is also true. In a recent demonstration by Sanger, insulin is monomeric and consists of two polypeptides. One polypeptide, chain A, is an acidic chain with 21 amino acids. The other polypeptide is a basic chain, B, with 30 amino acids (see figure 2 below).4 The two chains are joined together by two disulfide bonds. These bonds are situated at A7 and B7, as well as at A20 and C19. There is a third disulfide bond that links the cystein residues at positions A6 and A11.5 This additional bond is important because it determines the type of tertiary structure of insulin that will be formed. It also determines the binding property of insulin.
Bonding takes place between the amino-terminal helix (A1-A8) in the A chain and a carboxy-terminal helix (A12-A20). A central helix (B8-B19) is a feature of the B chain. In addition, it is bordered by extensions of amino- and carboxy-terminal strands. A T conformation thereby results from the arrangement of these A and B chains. On the other hand, an R conformation is formed when the B chains are attached to the N-terminus (B1-B19 instead of B8-B19).
Pharmacodynamics and Pharmacokinetics of Insulin
Binding of Insulin to Plasma Proteins
Apparently, there is no literature that articulately indicates binding of insulin to plasma proteins. However, a very old study investigated the binding of exogenous warfarin and lidocaine to plasma proteins. Insulin is not bound to plasma proteins, but it is bound to basic proteins like the receptors to allow its functionality. During fasting, insulin is released by the B cells continuously at an estimated rate of 0.5-1 unit/h. The secretion of insulin escalated by 3 to 10 folds after food intake; subsequently, totaling daily insulin secretion to 18-32 units.
Degradation of Insulin
The half-life of insulin following release is short, lasting around 4 to 6 minutes. Insulin clearance in the liver takes place as the hormone passes through the portal circulation. Under such circumstances, the levels of insulin in the systemic circulation are seen to be lower in comparison to those in the hepatic circulation. The kidney clears insulin passing through the systemic circulation, but there are clearance issues among individuals with kidney disease. Clearance of insulin by the kidneys takes place through two discrete routes. The first route is defined by glomerular filtration alongside subsequential endocytosis. Endocytosis is the “luminal reabsorption of insulin by proximal tubular cells.” The second route is characterized by diffusion of insulin from peritubular capillaries. In addition, insulin is bound to contraluminal membranes (mainly those that form a lining on the distal half of the nephron) of tubular cells.
The binding of insulin to the distal half of the nephron triggers various important processes. These processes include reabsorption of “sodium, phosphate and glucose.” On the other hand, insulin bound to the proximal tubular cells is reduced to oligopeptides and amino-acids. Unfortunately, the enzymatic pathways believed to be involved in this degradation process are yet to be articulately delineated. Presumably, one enzymatic pathway involves the actions of “either non-specific proteases or GIT and insulin protease while the other involves the GIT and lysosomal proteases.” Insulin degradation end products are reabsorbed, via diffusion, into the peritubular capillaries. 60% of endogenous insulin is cleared by the liver in non-diabetic individuals while the kidney eliminates around 35-40%.7 In the case of diabetic patients, the converse is the case. The figure below illustrates this metabolic clearance of insulin.
The liver is largely involved in metabolic clearance of endogenous insulin while the kidney is mainly involved in the degradation of exogenous insulin.
C-peptide, unlike insulin, does not undergo metabolism as it passes (first phase) through the liver. However, 70% of its clearance takes place in the kidney. C-peptide concentration in serum is a reflection of pancreatic release of endogenous insulin among individuals whose kidneys are functioning normally. During insulin metabolism by the liver, the first step is the binding of insulin to a receptor. The result is partial degradation of the insulin accompanied by release of byproducts into the incubation medium. Some insulin is internalized and degraded within the cells. The byproducts of insulin degradation by the liver are similar to those resulting from the sequential actions of insulin protease.
Rarely does insulin degradation result in insulin in urine. Any remaining insulin whose degradation cannot be accounted for by either the kidney or liver is degraded in the muscle and other insulin-sensitive tissues within the body.
Insulin regulation
Information on insulin regulation is mainly derived from rat models; hence, the reason for the minuscule information on this topic.
The beta (B) cell is where insulin is synthesized, and subsequently stored in secretion granules in adequate amounts to meet body needs. Higher requirements lead to increased insulin secretion, and the converse is also true. The insulin gene in humans is situated on chromosome 11 between thyrosinhydroxylase (TH) gene and the insulin-like growth factor 2 (IGF-2) gene.
Insulin is produced as a sequential activity of food ingestion, a process that defines glucose as the nutrient secretagogue. Glucose-induced insulin stimulation necessitates the uptake of glucose into the cells and metabolic degradation of the glucose. The biosynthesis of glucose is triggered by the insulin promoter gene and mediated by various transcription factors that bind to particular sites of the DNA molecule promoter. Glycolysis in the B cell stimulates insulin transcription, and simultaneously, insulin biosynthesis takes place through positive feedback when insulin is secreted.
Insulin is produced in a preproinsulin form that undergoes sequential processes to become insulin. First, a signaling peptide with 24 amino-acids stimulates the binding of the preproinsulin to endoplasmatic reticular membrane and permits the entry of the preproinsulin form into the reticulum. Peptidase catalyzes the split of the bound preproinsulin to release proinsulin. Proinsulin contains the insulin A and B chains joined by connecting or c-peptide.5 Availability of ATP, calcium, guanosintriphosphate and microtubules enables proinsulin transportation to the Golgi complex where it is distinguished from other secretion proteins and enzymes. The secretory granules that are insulin storage sites develop within the Golgi complex, and their maturation entails acidification, loss of clathrin, which is the coat for the immature secretory granules, and conversion of proinsulin, which relies on pH, into equal amounts of c-peptide and insulin.
Nutrients, hormones and neurotransmitters regulate the biosynthesis of proinsulin. The stimulation by glucose is reliant on threshold concentrations of 2-4 mmol/l, but secretion occurs when the glucose concentrations are at 4-6mmol/l. Maximum proinsulin biosynthesis halts when glucose concentrations are at 10-12 mmol/l. The growth hormone, glucagon, and glucagon-like peptide-1 (GLP-1) are involved in insulin biosynthesis. Catecholamines, on the other hand, inhibit insulin biosynthesis while somatostatin has no effect on this biosynthesis process. Pregnancy and obesity escalate proinsulin biosynthesis while starving and aging inhibit it.
Hormones Regulation of Insulin Secretion
The growth hormone controls insulin secretion through somatogenic and lactogenic beta cell receptors. Amylin cooperates with insulin in that both suppress the secretion of post-prandial glucagon. Subsequently, both hormones lower the levels of glucagon-stimulated hepatic glucose after food intake. Important to note is that in the event of insulin-induced hypoglycemia, “amylin does not inhibit glucagon secretion.” Amylin slows gastric emptying, which is the time between emptying of contents from the stomach and arrival to the small intestines for absorption. Incretin hormones, for example, glucagon-like peptide-1, enhance insulin secretion and inhibit glucagon secretion.
Nutrients Regulating Insulin Secretion
Vitamin D regulates insulin secretion via 1.25-dihydroxycholecalciferol receptors in beta cells. Calcium is important for proper insulin functioning through increased accessibility of ATP-sensitive potassium channels.
Insulin Receptors
Insulin receptors plug into the plasma membrane (membrane-bound), and are composed of “2 alpha subunits and two beta subunits joined by disulfide bonds” as shown in figure 3 below. The insulin is plugged into the cells, but only the beta cells manage to embed into the plasma membrane. The alpha chains, on the other hand, remain outside the cells. Apparently, these alpha chains, outside the cells, are the ones that hold the insulin binding domains.
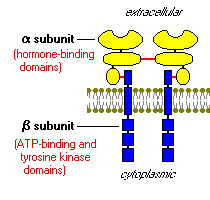
Insulin receptor: tyrosine kinase, acts in a similar manner to an enzyme by transferring phosphate elements from adenotriphosphate to tyrosine residues in intracellular target proteins. The binding of insulin to the receptor activates the catalytic activity of this receptor by autophosphorylation of the beta subunits. The insulin receptor has a catalytic activity that phosphorylates several intracellular proteins. These intracellular proteins further alter the phosphorylation process and a chain of biological response is created. An example of the phosphorylated intracellular proteins is the insulin receptor substrate 1 (IRS-1).3 IRS-1 acts as a docking center for identifying and activating other enzymes that regulate insulin activity.
Insulin in Diabetes
It is impossible to understand the role of insulin in diabetes without understanding insulin action, first.
Insulin Effects
Insulin is a critical agent in the regulation of intermediary metabolism by organizing the utilization of fuels through oxidation or storage. Therefore, insulin has great effects on the metabolism of both lipids and carbohydrates. In addition, protein and mineral metabolism is also greatly influenced. Elevated blood glucose levels stimulate the release of insulin, which subsequently stimulates the “uptake, utilization and storage” of this glucose by cells throughout the body. Depending on the target tissue, insulin has two major effects:
Entry into muscle, adipose and other tissues
Cells take up glucose through facilitated diffusion by hexose transporters and the main transporter is the GLUT4. This transporter is situated within the cytoplasmic vesicles, which fuse with plasma membrane when insulin binds to its receptors in target cells. The fusion process leads to insertion of the GLUT4 into the cells so that they can efficiently take up glucose. Low insulin levels result in recycling of the glucose transporters back to the cytoplasm. The brain and the liver are examples of tissues that do not rely on insulin for efficient uptake of glucose because they use a non-insulin dependent transporter.
Insulin stimulates glucose storage
Insulin activates the enzyme hexokinase in the liver with the aim of phosphorylating glucose and enabling glucose confinement in the cells. Insulin inhibits the action of glucose-6-phosphatase and activates several other enzymes involved in glycogen synthesis, for example, “phosphofructokinase and glycogen synthase.”
The two mechanisms discussed above explain insulin activity in a normal healthy individual. Low blood glucose concentrations are associated with reduced insulin secretions. When glucose, which is vital in the production of the body’s energy, is not accessible for the cells, alternative fuels, for example, fatty acids, are used.
Insulin and Diabetes
The World Health Organization classifies diabetes mellitus into “type 1 diabetes, type 2 diabetes, and gestational diabetes.” Despite the fact that all are attributed to the inability of the B cells to release adequate insulin, the causal factors are different. Type 1 diabetes results from the destruction of the pancreatic beta cells, type 2 diabetes is due to insulin resistance of target tissues resulting in high levels of systemic insulin. Subsequently, the beta cells are unable to meet the body’s insulin demands as a result of the resistance. Gestational diabetes is genetic, and resistance is brought about by pregnancy hormones.
Individuals affected by type 1 diabetes are typically healthy, and usually have a normal weight. The onset of the disease is marked by unaltered sensitivity and response to glucose. Type 1 diabetes is less common and account for around 10% of all diabetes mellitus cases. Insulin replacement, monitoring of blood glucose and a healthy lifestyle are paramount in the management of this disease. Type 2 diabetes is characterized by insulin resistance, and this is evident in the early stages of this disease. Central obesity is a causal factor for insulin resistance among individuals with type 2 diabetes. Abdominal fat is hormonally active, deemed to secrete the adipokines that presumably impair glucose tolerance. In the event that insulin deficiency results with time, exogenous insulin is necessary to maintain glycemic control.
Insulin and fat metabolism largely regulate insulin action in a manner that ensures the maintenance of acceptable glucose levels in blood. Insulin resistance is a paramount etiological factor of metabolic diseases and is linked to hyperinsulinemia. Poor insulin extraction in the liver leads to chronic hyperinsulinemia especially in obesity. Hyperinsulinemia due to insulin deficiency is associated with lack of the hormone amylin, which is secreted and located in the same region as insulin: beta cell hormone. Subsequently, postprandial glucose levels increase because of lack of insulin to stimulate “glucose disappearance, abnormal gastric emptying after food intake, and abnormal regulation of hepatic glucose production.”
In the case of diabetes and exogenous insulin is used, it is important to note that this insulin does not effectively suppress glucagon secretion; hence, blood glucose regulation becomes a great challenge resulting in postprandial hyperglycemia. Postprandial beta cell action is abnormal at the onset of type 2 diabetes due to the fact that insulin response after a meal is not realized. Due to peripheral insulin resistance in addition to dysfunction of the beta cells, the aftermath is unavailability of insulin, GLP-1, and amylin. Sequentially, hyperglycemia, which is a paramount symptom in diabetes, results.
Conclusion
It is evident that impairment of insulin biological activity leads to either hyperglycemia or hypoglycemia; thus, ensuring adequate levels of insulin in the body is important. Insulin therapy is administered to individuals whose bodies are not able to maintain optimal levels of insulin to allow glucose regulation in the blood. It is comprehensible from this paper that insulin dysfunction on its own is not adequate to cause diabetes mellitus; rather, it is an interplay of gastrointestinal hormones that has an influence on the pancreas. Unfortunately, insight into insulin synthesis, secretion, metabolism and degradation is limited due to unavailability of human subjects that can be used in this kind of academic project. Despite the fact that there is so much literature on diabetes, there is little literature on the hormones that actively regulate the occurrence of this diabetes. It is important for academicians and researchers to do further investigations of the metabolic events that define insulin metabolism, and how different disease conditions affect these metabolic events.
References
Mitchell, A.; Dick, R. Liver, gall bladder, pancreas and spleen. In Applied Radiological Anatomy; Butler, P.; Mitchell, A. W.; Ellis, H., Eds.; Cambridge University Press: Cambridge, 1999; p. 252-255.
Avolio, F.; Pfeifer, A.; Courtney, M.; Gjernes, E.; Ben-Othman, N.; Viera, A.; Druelle, N.; Faurite, B.; Collombat, P. From Pancreas Morphogenesis to β –Cell Regeneration. In Endocrine gland Development and Disease; Thomas, P., Ed.; Academic Press: San Diego, 2013; p. 218-232.
Bowen, R. Physiologic Effects of Insulin. Web.
Beta Cell Biology Consortium. The Structure of Insulin. 2015. Web.
Diapedia. Insulin synthesis, secretion and degradation. Web.
Iglesias, P.; Diez, J. Insulin therapy in renal disease. Diabetes, Obesity and Metabolism, 2008, 811-823.
Rabkin, R.; Ryan, M. P.; Duckworth, W. C. The renal metabolism of insulin. Diabetologia, 1984, 351-7.
Duckworth, W. C.; Hamel, F. G.; Peavy, D. E. Hepatic metabolism of insulin. The American Journal of Medicine, 1988, 71-76.
Goodman, H. M. Basic Medical Endocrinology, 4th ed.; Elsevier: California, 2009.
Skrha, J. Pancreatic Hormones and Hormonal Regulation of Insulin Secretion. MetaboČASOPIS LÉKAŘŮ ČESKÝCH, 2006, 599-605.
Diapedia. Insulin, glucagon and other metabolic hormones. Web.
Chalem, L. D. Thrive with Diabetes: Lead an optimistic, fun, challenging, fit, tenacious, enlightened, innovative & heroic life; BookSurge Publishing: London, 2008.